Wind Tunnel Studies for Flat Roof Solar Structures: What, How and Why?
5 June 2024
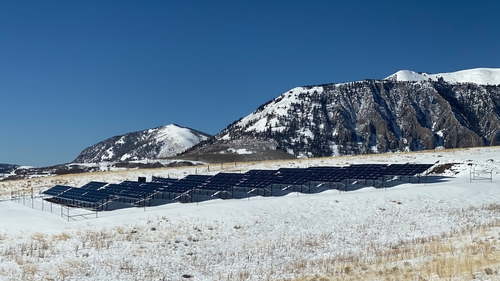
The US solar market has experienced a remarkable surge in installations over the past decade. In 2023 alone, “the US solar industry installed 32.4 GWdc of new capacity, a 51% increase from the previous year”. This growth has been particularly pronounced in the commercial sector, “which set a new annual record in 2023 with 1,851 MWdc installed, a 19% increase over 2022”.
Large, underutilized commercial rooftops present an attractive opportunity for energy and revenue generation. This is even more relevant for roofs having a reflective white membrane by using bifacial modules as they are even more profitable by lowering the building's thermal load input and maximizing the energy output. As a result, more and more building owners are opting to install solar systems on their rooftops. Installing a PV system on new or existing roof structures introduces interesting engineering challenges. One of the key constraints for such projects is the roof's load capacity. Ideally, the installation of solar panels should not significantly increase the dead load to the point where the roof structure requires modifications.
The added dead load for rooftop solar systems often comes from the use of ballast blocks, which are employed to counteract wind uplift and secure the solar installation to the roof. Ballast blocks are not the only restraint method available, as anchors or a combination of anchors and ballast can also be used. For ballasted systems, it is crucial to limit the additional weight on the roof structure as much as possible, in order to minimize costs and avoid overstressing the roof.
A wind tunnel study can play a crucial role in optimizing the ballast requirements and overall system design, thereby reducing the added dead load on the roof. Without such a study, designers are often constrained to using either general conservative models, such as those provided by ASCE 7-22 (Section 29.4.3), NBCC 2015, or SEAOC PV2 2017.
What is a Wind Tunnel Study?
The model is placed on a turntable that can orient it to different angles relative to the wind flow. The model is equipped with pressure taps on the top and bottom surfaces of the PV modules, allowing the net wind pressures acting on the system to be measured.
The wind tunnel parameters, such as flow speed and terrain roughness, are adjusted to match the required conditions stated by the ASCE 49. The model is then tested at multiple wind incidence angles, and the pressure data is collected as time series measurements.
Figure 1. One the left: side view of the array model mounted on top of a modeled flat roof building with visible pressure taps reaching the module surfaces. On the right: The global model mounted on a turntable permitting any orientation of the model in regards to the flow.
The results are post-processed and normalized to express them as equivalent static pressure coefficients (Cp). A common output format is an enveloping piecewise An-Cp function, where AN is the normalized tributary area and Cp is the associated worst-case pressure coefficient (both unitless). Enveloping curves generally considers the worst case (wind incidence, location within the array, etc) of a given tributary area (AN).
Figure 2. Obfuscated example of an AN-Cp curve. Data points represent all the peak pressures measured for all relevant configurations of adjacent PV modules. Grouping of surfaces are whole numbers of modules (example: 1x1, 1x2, 2x1, 2x2, etc.) hence the vertical alignment of data points. Finally the enveloping curve is a piecewise log-lin function that takes into account all extreme cases and provides a practical design tool.
Using the Results of a Wind Tunnel Study
Building Codes
The design of rooftop solar structures and their securement (ballast, anchors or both) is generally prescribed in building codes, such as the ASCE 7-22 and the NBCC 2015. These codes provide their own conservative AN-Cp curves, but they also conditionally accept the use of proprietary wind tunnel study results.
Regardless of which wind data is used (public or proprietary), a structure that is up to code requires the investigation of different loading scenarios (load combinations) to ensure all limit states are addressed. For example, the critical load combination for uplift resistance may differ from the critical combination for point loads on the roof structure. Failing to investigate all relevant load combinations can lead to designs that do not meet code reliability targets (see: Structure Magazine Dec. 2023 p.46 and SEAOC Statement about this important issue).
Tributary Areas VS Effective Wind Areas
The terms "tributary area" and "effective wind area" are both used in the industry for the design of wind uplift resistance, but their usage or interpretation can sometimes be ambiguous. This is how they should be interpreted, minimally in the context of rooftop solar structures.
Tributary Area (or Normalized Tributary Area)
This refers to the reference area for which the aerodynamic effect is rigorously defined by wind tunnel experts, as expressed in AN-Cp curves.
Effective Wind Area (EWA)
This is the derived equivalent or design area value used to justify the structural response as part of the forces counteracting wind uplift. These values are typically defined by solar mounting system manufacturers, and the determination methods can vary in rigor and quality.
Effective wind areas are often derived empirically through mechanical testing (pull tests) and/or calibrated numerical simulations (FEA). Currently, there are no official standardized methods for deriving these values, but the ASCE PV Solar Structure Committee is working on a Manual of Practice (MoP) which addresses this. In the meantime, it is recommended to seek a competent third party to assess the validity of the effective wind area determination method. Inadequate determination of these values can undermine the rigorous work of a wind tunnel study and lead to designs with reliability targets below those aimed for in the building codes.
Effective wind areas are sometimes interpreted as intrinsic property of a structure, such as material, but it is important to understand that it is not the case. They use AN-Cp curves to encode structural responses in a practical design value. Even if this approach can be conditionally and practically valid, these curves were not derived with this intent. In practice, depending on the determination method, effective wind areas can vary depending on the aerodynamic context (for example the position on roof) and magnitude. In the future, a simpler approach should be considered where aerodynamic effects and structural responses are kept separated in the design process and avoid obfuscation.
Limitations of Wind Tunnel Studies
Wind tunnel studies typically define their limitations in their reports. These limitations usually address the geometry of both the PV array (tilt angle, height, etc.) and the building (height, size, shape, etc.). It is crucial to respect these limitations when designing, as variables and magnitudes falling outside the study scope could lead to under-designed structures.
If a wind tunnel study yields design values significantly smaller than those published in building codes, it then requires independent peer review by another competent wind tunnel expert (ASCE 7-22 Section 31.5.3). In general, it is good practice to have a wind tunnel study peer-reviewed regardless of code requirements.
As science, engineering, and general knowledge on the aerodynamics of rooftop PV systems rapidly evolve, wind tunnel studies should be updated periodically. Wind experts recommend reviewing a wind tunnel study every three to four years to ensure it remains relevant and applicable. Stakeholders should be aware of this recommendation to avoid putting assets and the public safety at risk.
Conclusion
Wind tunnel studies are the industry standard for determining wind design values on rooftop PV solar structures. As our knowledge of the aerodynamic effects on these systems continues to evolve, it is crucial for all stakeholders to understand the key considerations when wind tunnel study results are used in a design.
First and foremost, the applicability of a wind tunnel study is clearly defined in their reports. It is the responsibility of the designer to respect the stated limitations on geometry, wind conditions, and other parameters. Blindly applying the results outside of the study's scope can lead to dangerously under-designed systems.
Additionally, wind tunnel studies should be viewed as living documents that require periodic review and updating. Experts recommend a thorough peer review and potential revision every three to four years to ensure the results remain relevant and aligned with the latest research and best practices. Stakeholders should consider this in order to protect their investments and the public's safety.
Finally, it is important to recognize that a wind tunnel study is just one piece of the puzzle. Adequate structural and ballast/anchor design depends heavily on the proper application of all relevant load combinations prescribed by building codes. Equally critical is the rigorous determination of "effective wind area" values, which should be peer-reviewed by a qualified third party to validate the methodology.
In conclusion, wind tunnel studies are an essential tool for designing resilient rooftop PV structures, but their value is maximized when used in conjunction with sound engineering practices, regular peer review, and a commitment to staying current with the rapidly evolving state of the art. By embracing these principles, we can continue to advance the adoption of solar energy while ensuring the safety and reliability of these systems for years to come.